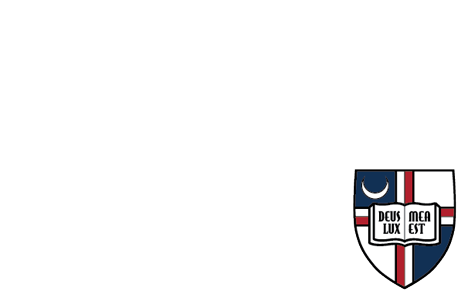
John Judge came to Catholic University as an assistant professor of mechanical engineering in 2004. He became associate professor five years later, and began serving as associate dean on June 1, 2016. Judge did his undergraduate work at Cornell and earned his Ph.D. at the University of Michigan, where he focused on vibrations in jet engines. In a postdoctoral fellowship at the U.S. Naval Research Laboratory, he turned to the study of microscale mechanical resonators, with applications in sensing and signal processing. Judge was appointed dean effective June 1, 2017. He took some time in July to discuss his vision for the school’s future and talk about his background.
What’s your vision for the engineering school’s future?
The school has seen a lot of enrollment growth in the last decade, in particular at the undergraduate level. The challenge we have going forward is to maintain the “small school” feel and the amount of personal attention students get. That’s a challenge when we’ve got twice as many students as we did a decade ago.
One of the things we would like to do is to grow the faculty. The undergraduate student body we’d like to keep stable — or if it continues to grow, make sure that it’s very measured growth — so we don’t outstrip our ability to provide a quality education.
Where we’d like to grow is the master’s programs. The typical model for a doctorate in engineering is that it’s funded in some way, such as by a faculty member’s research grant, whereas the master’s is a little bit more like undergraduate degrees, where students are expected to pay tuition. And it pays off — the bump in salary from having a master’s degree is worth the extra investment in tuition. In order to be able to maintain a good doctoral program, we need to have a solid base of undergraduate students and master’s students. The undergraduate enrollment has already gone way up over the last decade, so the place where I see room for growth is in the master’s program.
We have four departments [biomedical engineering, civil engineering, electrical engineering and computer science, and mechanical engineering], and historically they’ve been pretty siloed. I would like to see a lot more cooperation between the departments. There are areas of interest that cut across different disciplines. Environmental issues cut across different departments, whether it’s sustainable building practices and water treatment in civil engineering, or clean energy generation in mechanical engineering, or next-generation photovoltaics and power distribution systems in electrical engineering. These departments have all had their own efforts to address environmental issues, and I’d like to bring that together. Robotics is another area that cuts across many disciplines, and electrical engineering and computer science, mechanical engineering, and biomedical engineering can all contribute.
There’s a trend in higher education that I don’t like, which is the commodification of degrees, with incoming students asking, ‘How is this going to lead to a particular job?’ rather than ‘How is this going to broaden me as a person?’ At the same time, we are educating people for a particular career path, and we need to pay closer attention to what happens next. How are we placing our students into jobs? How are we placing them into internships while they’re still with us? I think we can do a lot more to increase our students’ professional preparation and give them the skills they need — not just solving equations, but working in teams and communicating; being able to write well; understanding how what they’re doing fits into what their company is trying to do, or how it might lead to a new product or even a new company. We want to educate people who are not just going to be good entry-level people, but who are going to rise to positions of leadership.
How do you see achieving this goal?
One of the things that we’ve always tried to do, and that we should do more of, is integrating what students learn outside of their engineering courses — in subjects like philosophy and ethics — into the engineering curriculum. Engineers who understand how what they’re doing fits into the bigger picture are more likely to wind up in positions where they’re in charge of the big picture. Being able to put things in context, being able to think ethically and morally rather than being narrowly focused on a technical solution — that’s something I think our school of engineering can provide in a way that’s stronger than other engineering programs.
All of our students have a capstone design project in their senior year. It’s supposed to tie together their engineering education over the previous three years and draw from things they’ve learned in all their classes, to put it all together. So one of the things that we need to work into these design projects is these bigger-picture ideas.
The National Academy of Engineering (NAE) has issued a list of 14 “Grand Challenges” for the 21st century. Catholic University is one of a small number of universities around the country to launch an NAE-approved Grand Challenges Scholars Program. One of the aspects of the program is that students are supposed to be thinking about these big-picture problems, and they work with a faculty mentor to do this, choosing their classes, internships, study-abroad options, and so on. Each student has an individualized plan related to one or more of these big issues, positioning them for a career addressing some of these problems.
Quite a few of the NAE’s grand challenges have to do with the environment and the planet and people’s relationship to it — finding clean sources of energy, giving the entire world access to clean water. There’s an interesting amount of overlap between what the National Academy of Engineering is suggesting are the big engineering problems for the 21st century and what Pope Francis is saying are things that we as a society need to do to maintain a healthy relationship with the world that we live in. The overlap between those is a really sweet spot for our school.
Your work with Engineers Without Borders seems relevant here.
I traveled with groups of students to Paraguay and to El Salvador. When I first joined the faculty here, I had a number of students that were interested in doing that sort of thing, and I had read an article about Engineers Without Borders, and it seemed like a really appropriate thing for Catholic University to get involved in. I’ve been the faculty advisor since it got started. The mission of that organization is to undertake sustainable engineering projects in the developing world. In El Salvador, we worked with the professional EWB chapter in D.C. and students from Howard and George Washington, and we wound up running water to 260 houses as well as building a cistern and a building that serves as the water office and community medical clinic. We’ve also had students travel to Panama with the D.C. professional chapter, and many of our students stay involved with EWB after graduation.
How did you get started in engineering?
When I was young, I thought about being an architect. I always liked drawing sketches of things and making plans for things. Somewhere along the line, it occurred to me that in some ways engineering is similar to architecture in that it’s designing and planning, but in mechanical engineering you’ve also got moving parts. I was drawn to mechanical engineering because it’s design but of things that are moving and interacting, and to me that was more interesting than static design.
My doctoral work was on vibration in turbomachinery. If you have a typical jet engine, you’ve got these rotating fan stages or compressor stages that have a bunch of blades. They’re like your desk fan, except for instead of three blades they’ve got 30 or 60 blades, and they spin at much higher speed. And in principle all of those fan blades are identical, but in practice they’re slightly different. It turns out that very small differences between the blades cause huge differences in the way the system behaves. In the normal course of events, you expect if you make a small change here, it leads to a small change there. It’s really interesting when a small change here leads to a huge change somewhere else.
The term “butterfly effect” gets used sometimes in these kinds of situations — it’s a reference to the idea that a butterfly flapping its wings in one part of the world could affect the course of a hurricane on the other side of the world weeks or months later. This sensitivity to small changes is a common occurrence in nonlinear dynamics, which is another one of my areas of interest and research. It’s really cool to study these problems and figure out why changing a little bit over here changes a lot over there. To come at that from a 30,000-foot view, that’s where you have leverage to make a difference. If I can understand the system well enough to make just a small change here, and achieve a big outcome with relatively modest changes on the input side, that’s a lot of bang for the buck.
Is it a form of optimism to focus on small tweaks leading to big changes?
Whatever system you’re talking about, whether it’s a human-built engineering system like a jet engine, a social system, or a natural system like the weather, optimism comes from the idea that if we can understand how it works, we can find aspects of the system which give us the leverage to have a big effect with a relatively small effort or change. Basically, at some level, that’s one of the things that engineering is all about. It’s not pure science. Science is understanding how the world works just for the sake of knowing. Engineering is understanding how things work for the sake of being able to change them. It could be civil engineers building a building that’s not going to fall down, or mechanical engineers building a vehicle that’s going to get you from point A to point B, or electrical engineers building a communications system, or biomedical engineers designing a medical device. It’s understanding the scientific principles behind how the world works with an aim in mind, which is to build something or accomplish something that’s going to make a difference in people’s lives.
You went from studying this on a big scale to microscale.
When I came to the Naval Research Lab after graduate school, I started doing work on micromechanical systems. I was applying some of the same principles that I had been studying in graduate school to vibration of devices at very small scale. For example, the filter that is in most people’s cellphones is a mechanical device. The antenna picks up the radio waves and the cellphone has to take signal from the antenna and filter out everything else except for whatever frequency is being used for that communication. This applies to any sort of wireless communication — you’re picking up whatever’s floating in the air, and you need to filter out everything except the channel that you’re interested in. You would think that filtering would be done electronically; it’s actually more commonly done mechanically. The electrical signal from the antenna is converted into a vibration on a very small mechanical device. The device sets up a vibration at one side and then at the other side of the device converts that vibration back into electrical signal. So it’s a mechanical filter inserted into an electrical system. It’s easier and cheaper to make it work well with mechanical components. The vibration research that I was doing in jet engines turns out to be applicable to completely different applications.
Having done research at both large and small ends of the spectrum, what are your conclusions?
Basically, any time you’ve got a system of things that are supposed to be identical and it turns out they’re not quite identical, it turns out there is a potential for that to have a huge impact on the way the thing vibrates. There are a lot of examples of that throughout different fields of engineering, where things that are nominally identical aren’t quite identical, and sometimes it makes a huge difference.
True identical likeness is rare. In a lot of cases, it doesn’t matter. If you build a bridge and the spacing between the support structures isn’t perfectly identical, it probably doesn’t make the bridge fall down. If one column is half an inch off from where it should be, it’s not going to have a big effect. The interesting thing is to identify those problems where a small tweak actually has a surprisingly huge effect on the behavior of the system, and to be able to watch out for it, if it’s a surprisingly big negative effect the way it is in jet engines. But there are other applications where introducing just a little bit of difference gets you a positive effect that you want.
It’s interesting to me that the same physical and mathematical principles that apply in large structures like a jet engine can be used in a completely different application, on a completely different scale. You can use some of the same mathematical and physical principles for a totally different application.
Our ability to build things at micro- and nanoscale is growing by leaps and bounds. The whole field didn’t exist 50 years ago. Then, you built things on a scale that you could see, and now we have all sorts of technology for building things on a microscopic scale or even on a nanoscale, even down to the scale where you’re actually assembling individual molecules and chains of molecules into the device. Micro- and nanoscale engineering is a fascinating field, and it dovetails and capitalizes on a lot of the advances made in electronics. All of our electronics are vastly more powerful now than they were when we were kids. It’s all advances in manufacturing at very small scale: How many transistors can you pack into a computer chip? For decades now, chips have been getting faster and more powerful, and the components smaller. Micro- and nanoscale fabrication of mechanical devices has the same potential to do really impressive things with really small systems.
I’ve also been doing acoustics research. It’s related to the other stuff I was talking about, which is understanding and controlling how vibration propagates through a structure, except for instead of talking about vibration of solid structures, we’re talking about vibration of the air or water and the propagation of that energy from one point to another.
One thing we’ve been looking at is altering the acoustic properties of a space by making small changes. You’ve got the engine that’s vibrating and shaking the frame of your car, and it’s loud inside. Same thing in an aircraft or any other sort of vehicle — you want to minimize the noise and also minimize the vibration itself. If you’re sitting in the wrong place in a vehicle, you can feel the engine vibrating. Forget about hearing it, you can feel it through the frame of the vehicle. Making small tweaks either to the structure or, in the acoustics example, to the way the space is designed can have a big impact on what you hear or what you feel.
Your wife is also an engineer. Do you have professional debates at the dinner table?
She teaches at the Naval Academy. We have lovely professional conversations at the dinner table and the kids just roll their eyes. We have three children, one each in high school, middle school, and elementary school. We don’t know yet whether they will wind up as engineers or flee, screaming, in another direction.